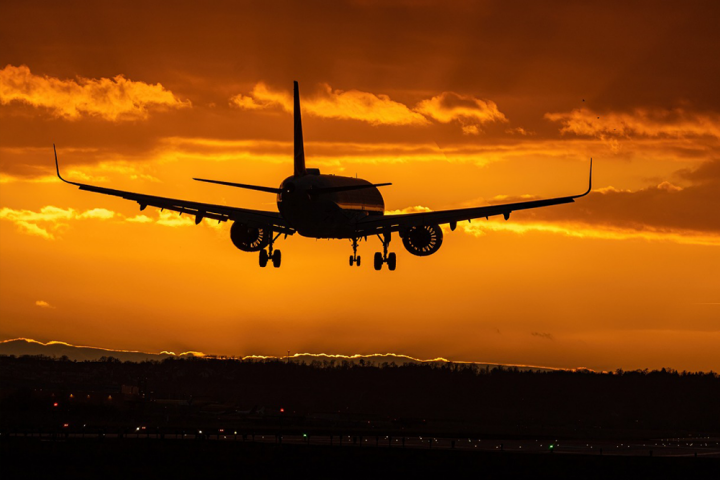
Composite materials at full throttle
Despite often being considered cutting-edge, using composite materials is nothing all that new in the world of aviation. In fact, the aircraft industry could even be hailed as a pioneer in the field as it was one of the first industries to use such materials on a large scale, despite their cost being higher than the metals they replace. Ever since the Wright brothers and Clément Ader created their first “flying machines”, aircraft manufacturers have understood that weight is a key consideration when it comes to successfully getting a plane in the air. This was certainly true back in the days of the first aviators; however, modern engines are so powerful that it is now much easier to get heavier aircraft to take off. Nowadays, the pursuit of lightweight structures is undertaken with other goals in mind. One of these is economic efficiency, as lighter aircraft consume less fuel. This is linked to another key goal, energy efficiency since less consumption means less greenhouse gases.
![]() A 12 HP engine, a few planks of wood and some cotton canvas with a total weight of 272 kg… Who would have guessed that a plane would break the sound barrier just five decades after the Wright brothers’ great feat in 1903… |
Polymers pull their weight
Composite materials are so named because they consist of two or more elements: a fibre (fibreglass or carbon fibre, for example) and a matrix. A plastic resin bonds and holds the fibres together, ensuring the materials’ integrity and enabling the transfer of mechanical loads between fibres. These materials first appeared in the world of aviation back in the 1930s, when a combination of fibreglass and resin was used to reinforce propellers. These reinforced propellers were stronger, but this new material was not really any lighter than wood. It was not until the 1970s that new composites emerged, usually based on carbon and epoxy resin.
![]() Polymer resin-based composite materials have put aircraft on a diet. Being lighter than the alternatives, planes that include these materials consume less fuel. Here, a composite panel is fitted to a thrust reverser. |
These high-tech materials were full of promise: they were lightweight but highly resistant, particularly to fatigue, and unaffected by corrosion and, more often than not, chemical attack. |
The only drawback was that they were not easy to use: moulding was a tedious process that required numerous manual operations, and the ideal heating times to harden them properly were based on empirical techniques. Essentially, the material was promising, but it still needed taming. Through hard work and perseverance, engineers have succeeded in further mastering these materials and bringing them in line with the strictest aviation standards. Today, they account for 50% of the weight of certain wide-bodied aircraft. Boeing was the first to achieve this game-changing 50% on its 787, which received its certification (i.e., its licence to fly) in 2011. This was a real feat considering its competitor at the time, the Airbus A380, only just contained 25%. Not wanting to be outdone, the European manufacturer introduced the A350 a few years later—and this time, it exceeded the 50% mark. On these aircraft, the fuselage, tail, and part of the wings and ailerons are made entirely of carbon fibre panels and epoxy resin. We have made incredible progress in just 50 years, especially when you consider that the Boeing 747, the “it” plane of its time, garnered a lot of publicity for its aluminium alloy design…
Composites from nose to tail
These materials attract attention predominantly for their weight. However, aircraft manufacturers are also interested in composites for their other lesser-known qualities.
Their unparalleled rigidity, for example, is ideal for manufacturing large parts such as wing components. Their moulding manufacturing process also reduces the number of parts assembled by screwing or riveting that need to be closely monitored and replaced if there is the slightest issue. That is a lot less maintenance, especially as these materials are unaffected by humidity and corrosion, unlike metals. |
![]() Composites are not just lightweight; they can also be moulded as required, making it possible to manufacture parts in a single piece where several would have been needed for metals. |
Moving parts within the tail and ailerons are made from the same material, though here, it takes on a sandwich structure. This type of construction means the composite plates can better resist torsional movements. It consists of a honeycomb core (a structure that minimises the amount of material required while still achieving maximum strength) made from Kevlar®, an aramid fibre, sandwiched between two layers of carbon fibre composite. The same technique is used for the radome, the convex tip of an aircraft’s nose. From the outside, this looks like a simple “end cap” that many might imagine serves solely aerodynamic purposes. However, this is not its only function. Hidden behind this “cap” is the radar (in particular, the vital weather radar that is capable of detecting large clouds). To work effectively, the radar must be able to pass electromagnetic waves back and forth. Polymers are particularly effective in this regard, though they are less suited to the high impacts, especially from birds, that radomes are exposed to. The honeycomb construction makes them virtually indestructible. In most cases, they are also enriched with copper wire to dissipate any lightning strikes.
![]() The composite materials used to manufacture the Airbus A350 account for more than 50% of its mass. A first in the history of aviation. |
Composites: flexible solutions
The area connecting the wings to the fuselage is made of fibreglass composite, which is renowned for its robustness. Only the leading edges of the wings and engine mounts are metal: the former is made from aluminium, the latter from steel or even titanium. These are still top materials in terms of their impact resistance, but for how much longer? Airbus now reinforces part of the A320’s wings with aramid fibre, a fibre that is resistant to just about everything! Boeing will equip the upcoming 777x with composite folding wings—proof, if it were needed, of the maturity of composite materials. This is a daring choice by Boeing, but one that has been certified. With a wingspan of 72 metres, this giant aircraft risked hitting other planes on the stands. To solve this issue, these wings will fold up when the aircraft is on the ground, giving it a 7-metre wingspan while remaining perfectly stable in flight. The metal hinge system coupled with the composite wings is a winning combination, and one that utilises the very best of both materials.
![]() For the 777, Boeing has taken on the challenge of creating a folding wing. They have successfully risen to the task by using a combination of composites and metals. |
The polymer film festival
There are a few drawbacks to composite materials; however, not least their poor ability to conduct electricity and discharge it in the event of a lightning strike. To remedy this, a copper wire mesh is usually inserted into the fuselage.
![]() To dissipate electricity in the event of a lightning strike, some aircraft can be fitted with an adhesive polymer film incorporating copper wires. |
Solvay has even designed a surface-adhesive polymer film that can incorporate a very fine mesh of copper wires to dissipate electricity. |
In a similar vein, Lufthansa, in collaboration with BASF, has designed a polymer film inspired by shark skin. Just like the skin of the fish that inspired it (which is well known for its hydrodynamic capabilities), it is comprised of millions of prism-shaped riblets, each 50 microns (0,005 cm) deep. This ribbed texture reduces air friction on the fuselage, therefore reducing drag. 950 m² of film applied to an aircraft saves 400 tonnes of aviation fuel and 1,200 tonnes of carbon dioxide a year. If the global fleet of long-haul aircraft were fitted with this coating, nearly 5 million tonnes of fuel would be saved every year.
Plastics have got it in the tank
Thermoplastic polyimides (TPI) and, more specifically, polyetherimide (PEI), are mainly used in aviation in certain engine parts subject to gas exchange. Injection moulded, polyetherimide is lighter than metals such as aluminium, which it replaces. Its high resistance to high temperatures (over 150°C), chemical attack, hydrolysis and friction makes it a material capable of great feats.
This polymer family is compatible with high temperatures. Other high-performance thermoplastic materials, such as polyaryletherketone (PAEK) and, more specifically, PolyEtherEtherKetone (PEEK), are also found in landing gear grease nipples. This material resists pressure just as effectively as metal, so in this case, the aim is to offer a lightweight alternative. |
![]() High-performance polymers such as PEEK are now found in a number of places, including jet engines. The benefit? They can withstand anything, especially high heat. |
Epoxy-based polymers are used in areas at the front of jet engines to reduce fuel consumption. Here, achieving a lower mass is not the concern; instead, it is a question of the material’s abradability. An abradable coating is one that, when brought into contact with a moving part, wears down preferentially to said part. In jet engines, they are found on turbine seals. Reducing the clearance between moving parts (such as blades) and their casing to a minimum improves the aerodynamic performance of the engine, therefore improving its efficiency. Any friction will wear down the abradable seal without significantly affecting blade efficiency. There is now talk of placing new 3D-printed parts made from these polymers near the fans to reduce engine noise. This polymer can be transformed into a metamaterial of the micro-scaffolding type (an architectured material with a porous microstructure) with high-level acoustic absorption qualities through additive manufacturing.
By using different polymers such as PEEK and carbon/epoxy composite for the blades, CFM’s LEAP engine, which powers around 90% of the single-aisle medium-haul aircraft sold worldwide, is over 450 kg lighter than the previous generation, which weighed almost 2.500 kg. While these figures are significant, the real accomplishment is that this has reduced fuel consumption by around 30%.
![]() The latest generation of engines, such as the LEAP, are 20% lighter than previous generations thanks to polymers. |
Interior amenities: plastics feeling the heat
Overhead compartments, seat covers, service trolleys, partitions, walls, etc., polymers play a major role when it comes to aircraft interiors. There is a wide range of polymers available, from polyamide (otherwise known as Nylon®) to polycarbonate, polyetherimides (PEI) and even traditional fibreglass/epoxy composites. As in the automotive industry, these materials are primarily chosen for their light weight and ability to take on any shape. However, safety standards are even more strict in the air, particularly when it comes to fire resistance. To pass the necessary tests set out by the various certification bodies, materials must have excellent fire resistance. This is an inherent quality of PVC, which is used to insulate cables. Polyetherimides (PEI) also have high heat resistance, so logically they are found in larger parts such as partitions and other walls. For smaller parts, such as fastening systems, polyamides additivated with flame retardants are the plastic of choice. As for the overhead compartments, they are made of fibreglass and epoxy composite, an indestructible material that is ideal for objects that will be used thousands of times over their lifespan.
![]() Cabin interiors are now a key differentiating factor for airlines. Polymers are predominantly used because they are lightweight, but their design and texture can look the part too. |
It is the small details that make all the difference. PEI can also be enriched with carbon fibre to make it even more resistant. This plastic will replace the aluminium previously used in armrests, footrests, coffee makers and tray table arms, resulting in a few dozen kilograms fewer CO2 emissions or more cargo…
Putting plastics in the driver’s seat
While seat frames are still mainly made of metal, usually aluminium or titanium, the rest is made of various polymers; for example, you will find polycarbonate in the armrests and seat covers. Manufacturers are still trying to make seats lighter while complying with safety standards. A young French company has risen to this bold challenge, developing a seat with a titanium and carbon fibre frame. It weighs just 4 kg, substantially lighter than the conventional 10 kg seat. A true innovation, this is the first carbon fibre seat to be certified by the aviation authorities. This seat has recently entered the Airbus A320 and the Boeing 737’s catalogues.
![]() Using polymers in seating is nothing new. For a long time, seat frames were made of metal, but today there are ever-lighter designs incorporating composites. |
In the near future, plant fibres such as flax could be used in trolleys, for example. Aside from being environmentally friendly, they absorb sound and vibration much better than their carbon and metal counterparts—an undeniable benefit when considering the comfort of passengers and flight staff…